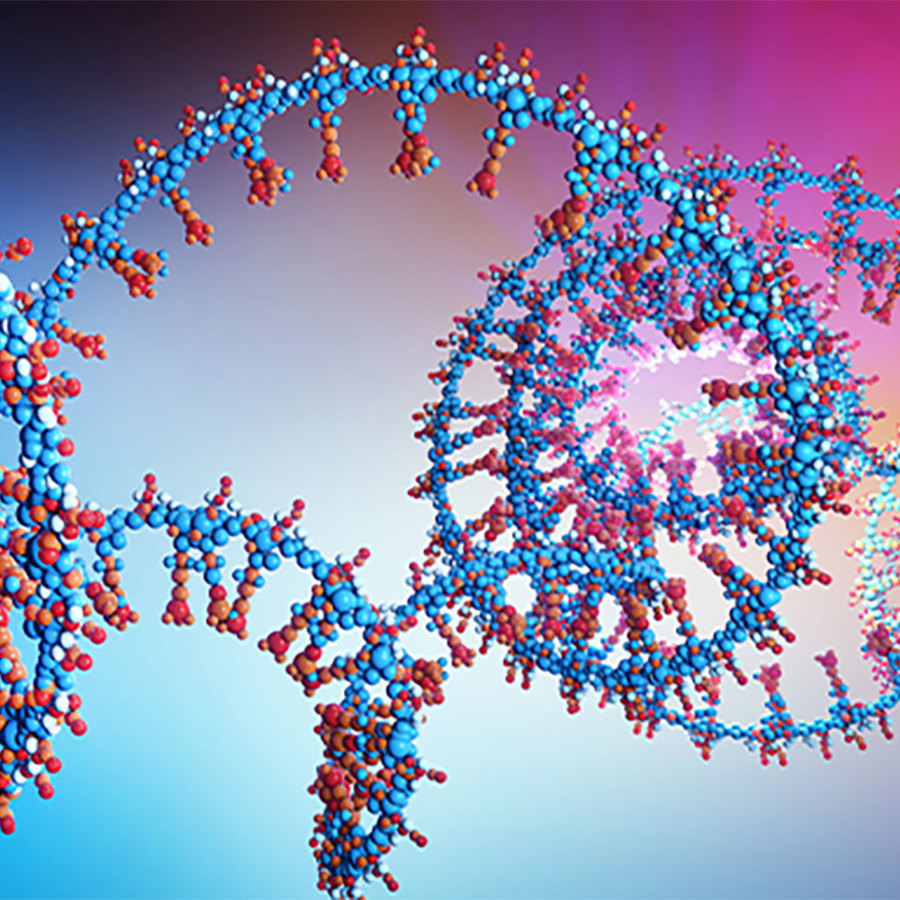
Does “junk DNA” have a specific purpose?
January 24, 2013
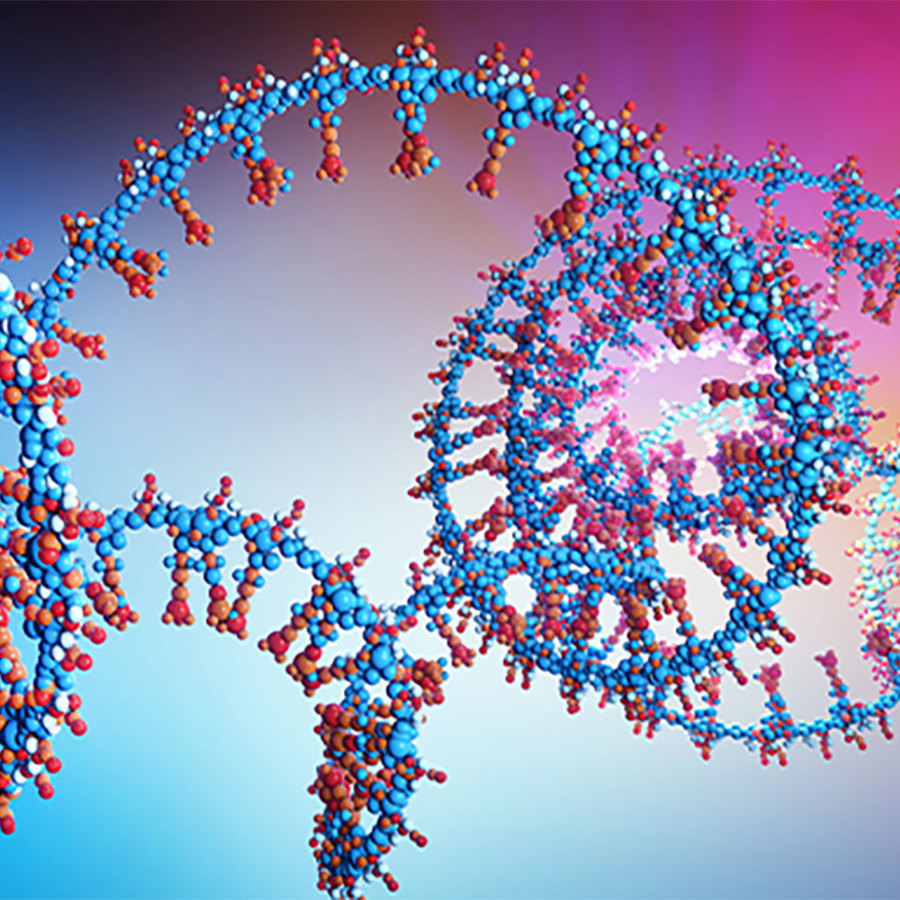
- Related Topics:
- DNA basics,
- Noncoding DNA,
- RNA,
- Genes to proteins
A curious adult from California asks:
“Does “junk DNA” have a specific purpose?”
Most of the time when people talk about “junk DNA,” they mean DNA that doesn’t have a function. In other words, it’s DNA that does not have a specific purpose.
It turns out we were too quick to call this DNA junk. We have discovered a lot of functions for DNA we didn’t know about before. In fact, it seems most “junk DNA” does have a purpose.
We now know that a lot of “junk DNA” is around to tell our bodies when, where, and how much to turn on a gene. New research tells us that most of the rest of the junk probably does unknown but important things too.
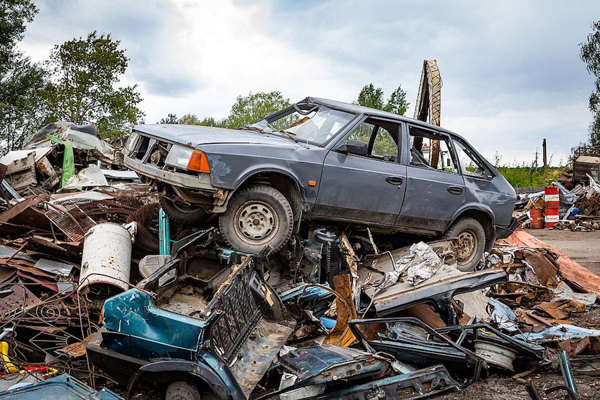

When we talk about DNA we usually focus on genes. This is because genes are the part of DNA that carries the information to make proteins. Proteins are the chemical machines that do everything in your body from making the pigment in your skin, to causing your heart muscles to contract.
The interesting thing is not that much of your DNA actually carries the specific information for proteins. In other words, not a lot of your DNA is taken up with genes, or coding regions. When the human genome was sequenced in 2001, scientists found that only about 3% of human DNA was genes (coding regions) and the rest was noncoding DNA.
This might make more sense if you think of your DNA like a cookbook with all the recipes you will ever eat. The genes are like the ingredients you need to make a recipe.
But most of the cookbook isn’t the list of ingredients; it’s the instructions of what to do with them. What temperature to use, when to add certain ingredients, and so on. This is the information found in all of that junk DNA.
Even though we were wrong about most DNA being junk, there probably is some DNA you don’t need. We know that some DNA can be deleted in mice without causing any apparent damage, and we think that might also be the case in humans. And there’s probably still some DNA that does stuff we don’t understand yet.
Turning on genes
Each and every cell in your body has all the DNA instructions needed to make you. But not every cell in your body does the same thing. Your muscle cells don’t do the same thing as your skin cells even though they have the same DNA.
What your cells have to do is only read the instructions relevant to that kind of cell. Muscle cells only look at the “muscle cell” part of DNA and ignore the rest. This way you don’t end up with a muscle cell that has hairs growing from it.
If we think about our cookbook, on any given day you only want to cook a few certain recipes. So that day you only look at the pages with those recipes. The recipes are like the genes a particular kind of cell needs.
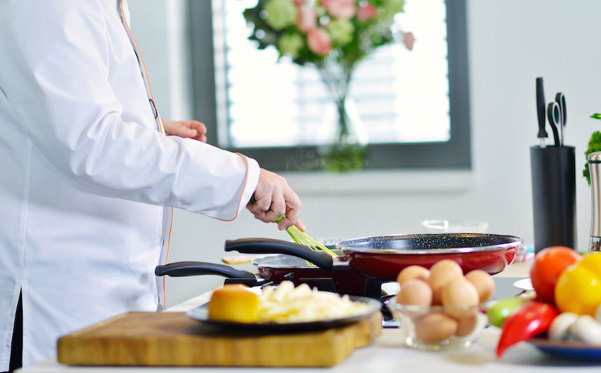
Let’s do a quick review of how DNA stores the information needed to make a protein. DNA is a code written in 4 chemical letters: adenine (A), guanine (G), cytosine (C), and thymine (T). The order of these letters tells a cell what amino acids to put together and in what order. Those amino acids are what make a protein.
But genes, and the proteins they make, are just the ingredients in our recipes. The instructions for when, where, and how much of those ingredients to use are mostly outside of our genes.
A lot of regulation of genes happens in front of coding regions. These parts of the DNA are called promoters.
Promoters carry a lot of information that tells the cells when, where, and how much of the protein to make. But that’s not the only part of DNA that controls making of proteins.
There are also other regions of DNA that contribute to when and where a protein is made. These regions don’t always have to be right in front of the gene which sometimes makes figuring out what piece of DNA regulates a coding region difficult.
To make things more complicated there is another molecule in the mix besides DNA and proteins. RNA is needed too.
mRNA and Introns
One of the things RNA does is copy the code from DNA and then act as a go-between between DNA and the machines (ribosomes) that put the amino acids together. This kind of RNA is called messenger RNA or mRNA. We’ll talk about other kinds of RNA in a little bit.
Going back to our cookbook example, if DNA is the cookbook then mRNA is a copy of a recipe. This way you don’t need to drag the whole cookbook around with you while you’re cooking.
What’s weird is that when DNA from a gene gets made into mRNA, not all of that mRNA gets used to make proteins. These pieces of DNA, that interrupt coding regions, are called introns.
Introns are cut, or ‘spliced,’ out of the mRNA before it gets translated into a protein. In other words, they aren’t used to make the final protein product.
At first introns might look like junk, but a lot of them are not. Some introns make their own small proteins. Others control how much of the protein gets made. And some are important in making different proteins from the same gene (alternative splicing). As you can see below, a cell just cuts out the introns in different places:
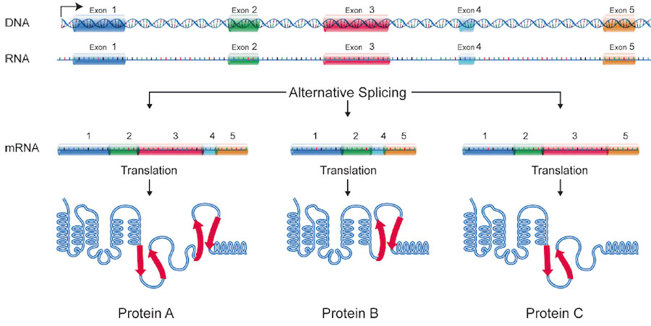
New studies have shown that about 80% of our DNA is made into RNA. But only 3% or so of all DNA has the information to make proteins. What is all that other RNA doing?
Noncoding RNA
RNA is a pretty amazing molecule. Not only can it carry the information from DNA but it can also act a little like a protein.
Even though RNA is a helix (but a single helix, not a double like DNA), it can fold into other structures. These structures can do work in the cell like a protein. There are quite a few things RNA can do besides carrying DNA’s message.
Different kinds of RNA are important in making proteins. The ribosomes, which put together amino acids to make proteins, are made up of a combination of RNA (called ribosomal RNA, or rRNA) and proteins. And the molecules that bring the amino acids to the ribosomes, called transfer RNA (or tRNA), are made of RNA as well.
Some smaller RNAs can match up with parts of mRNAs and cause that mRNA to be cut up before it ever gets to the ribosome. This means that even when an mRNA is made, it doesn’t always mean that protein is made. These RNAs can fine-tune the amount of protein made by changing the amount of mRNA that gets to ribosomes.
There are many other types of RNA molecules, of all different sizes, that are encoded in what used to be known as “junk DNA.” And most of these appear to regulate the coding regions of DNA in a lot of different ways.
That takes care of what most of the 80% of our DNA is doing. What about the other 20%? Turns out that a lot of this DNA is probably the leftovers of old genes and viruses. But even some of this DNA might be useful.
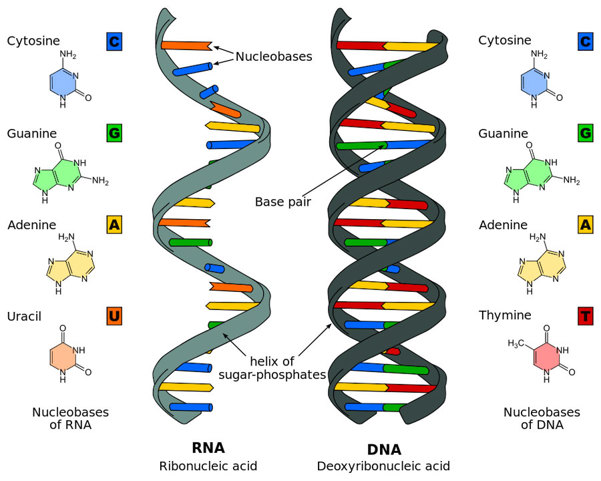
Leftovers
Some “junk DNA” could be left over from our evolutionary past. It used to do something but that job was taken over by some other piece of DNA, or we don’t need that job anymore.
Viruses also leave behind DNA. Many times viruses will insert their DNA into our DNA to make our cells do all the work for them. When the virus leaves the cell they often leave behind pieces of their DNA.
Sometimes this leftover virus DNA has a whole gene on it and that gene gets used in our bodies. But a lot of times the virus leaves behind just a part of a gene or a gene that doesn’t work. This DNA doesn’t do anything but still gets copied for the next generation.
Some of these old genes and virus scraps might not be doing anything now but could be useful in the future. Changing these old pieces of DNA is one way to make new genes!
There might be some true junk DNA in our genome. But we don’t know what pieces of DNA have functions we are just waiting to discover.
What we do know is that what we thought was junk DNA just a few years ago turns out to be useful. It ensures that mRNA and proteins are made only in the right cells at the right time in just the right amounts. So it might be that in a few more years there will be even less mysterious “junk” in our genomes.
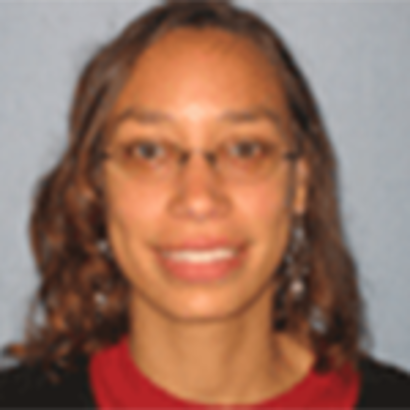
Author: Alisa Lehman
When this answer was published in 2013, Alisa was a Ph.D. candidate in the Department of Biology, studying nitrogen fixation and symbiosis in Sharon Long’s laboratory. Alisa wrote this answer while participating in the Stanford at The Tech program.