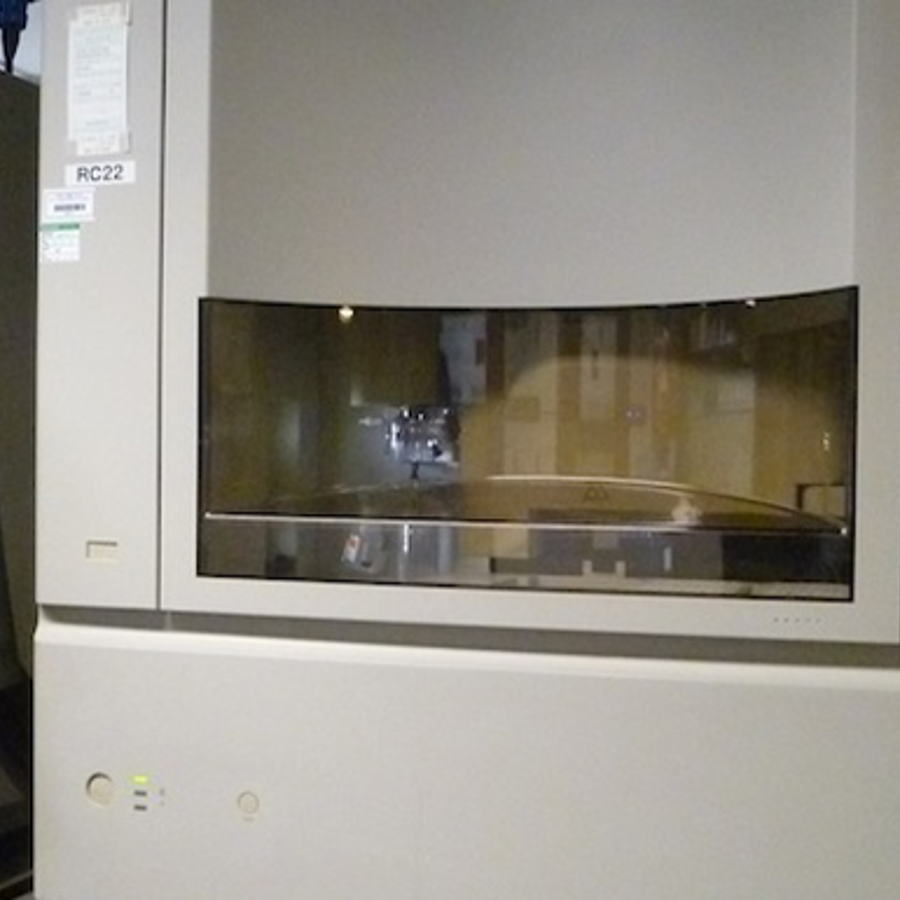
How does a gene sequencer machine work?
March 6, 2009
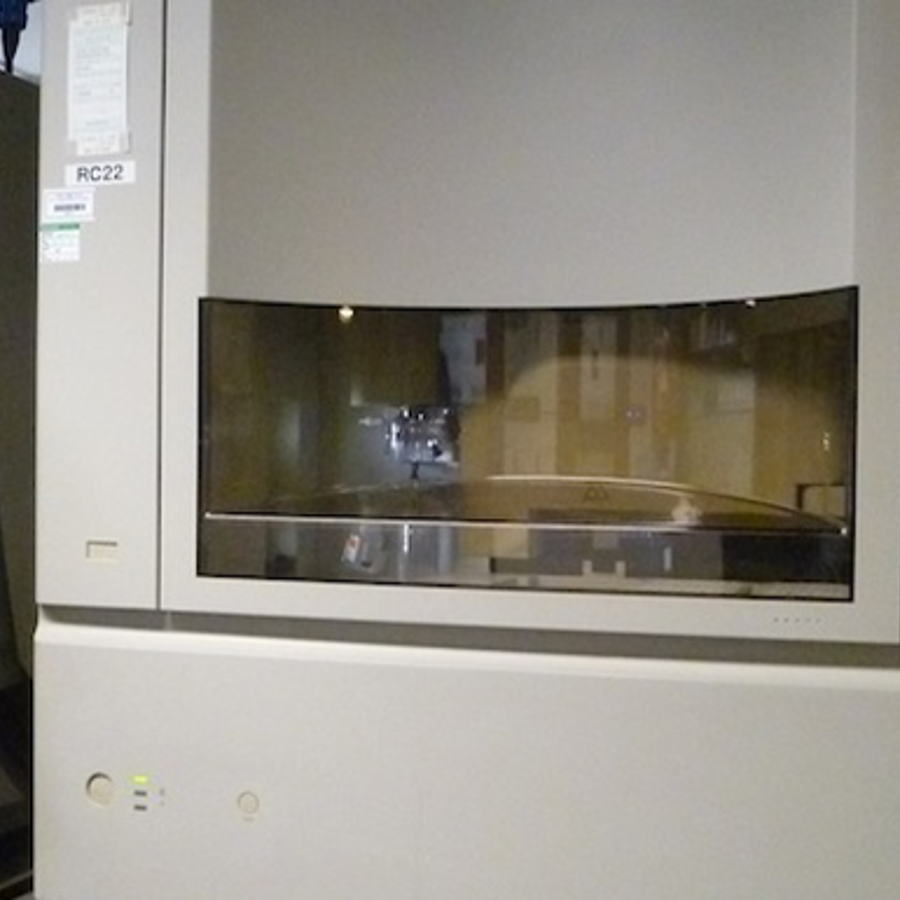
- Related Topics:
- DNA sequencing,
- Biotechnology,
- Forensics
A curious adult from California asks:
"How does a gene sequencer machine work? How are the genes in a genetic sample detected? I’ve seen these things used on TV, CSI et al, but I have no idea how they work."
Great question! Crime scene investigators and forensic scientists do use gene sequencers in their work. But they aren't sequencing genes. They are using gene sequencers to compare the size of certain parts of people's DNA. This lets them tell people apart and maybe catch a criminal.
I thought I would answer your question by first talking about how forensic scientists use part of a gene sequencer to catch criminals. Then I'll tell you about how scientists use a sequencer to read DNA.
As you'll see, scientists have to use a lot of tricks to be able to tell us apart cheaply and easily. And they have to use even more tricks to carefully read our DNA.
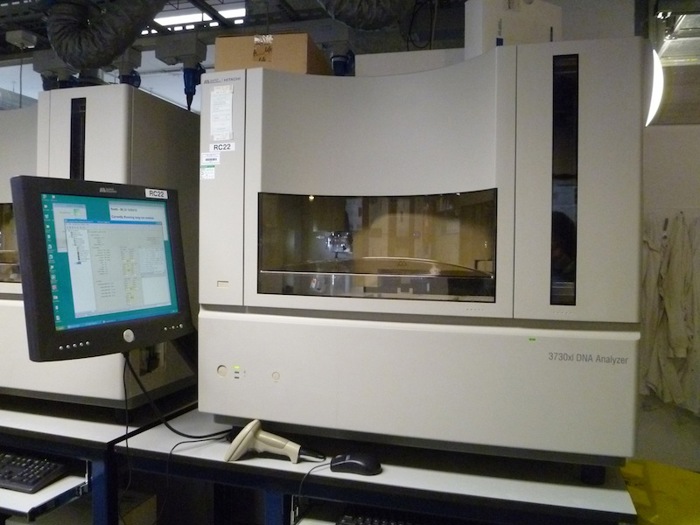
Short Tandem Repeats act like DNA Fingerprints
As I am sure you've heard, everyone's DNA is a little different. But what you might not realize is that everyone's DNA is pretty similar too. In fact, everyone's DNA is about 99.5% the same.
Human DNA is way too big to look at all of it for every person suspected of committing a crime. So scientists need to focus in on spots that tend to be different. The spots they've settled on are called short tandem repeats or STRs.
STRs are chunks of DNA that are repeated over and over, back to back. Remember, DNA is made up of four chemical "letters" (or nucleotides) that are usually abbreviated as A, G, T, and C. So an STR is a string of letters that is repeated over and over. What makes them useful here is that different people can have different numbers of these repeats.
Imagine the set of DNA letters AGTCCGTA. One person might have 10 of these in a row. And another might have 11 or 9.
Of course, lots of people will have 10 of these repeats. That is why scientists can't look at just one STR. Here in the U.S., forensic scientists have chosen 13 of them to tell people apart.
This actually gives scientists 26 pieces of DNA to look at because everyone has two copies of each of the 13 STRs. One copy comes from a person's dad and the other from a person's mom.
The lengths of these 13 pairs of different STRs make up a person's DNA fingerprint. The chance of two people having the exact same DNA fingerprint is at most 1 in a billion!
So scientists can tell most people apart using these 13 different STRs. To do this, they need to figure out how big the individual STRs are in people. This is where the gene sequencer machine you were asking about comes in.
Scientists use PCR to Pull STRs out of DNA
The first step in figuring out the length of a person's STRs is to pull the STRs out of the person's DNA. To do this, scientists use a process called PCR.
In PCR, you use a special protein called a "polymerase." Its job is to copy DNA. You tell the protein what DNA to copy by giving it starting and ending points, called "primers."
Primers are small segments of DNA that match the part of the DNA you're interested in. The protein then copies everything between the two primers. For us, this means we have to make a primer that matches on either side of an STR. The process would look something like this:
If someone has a different number of repeats, then they'll have a different size of copied DNA. Imagine our example above. If I have 10 copies of AGTCCGTA and you have 11, then my copied piece of DNA will be 8 letters shorter. The next trick is to see this DNA and to see the differences.
Scientists use Fluorescence and Capillary Electrophoresis to Size DNA
DNA is invisible to the naked eye. One of the things scientists do to make it visible is to add something fluorescent to the primers they use for PCR. Now the DNA will glow a certain color under the right light. Using PCR, scientists can make enough of this DNA to see the color.
Next though, they need to separate out the sizes. The way they do this is to run the DNA on something called a capillary electrophoresis system.
This is basically a material with a lot of different sized holes in it. The bigger the piece of DNA, the slower it moves through these holes.
Think about it this way. Imagine a coral reef with lots of different sized holes in it. Which is going to get through the coral faster, a small or a big fish? The small fish because it has more hole options to go through.
The same thing works with DNA too. The bigger DNA takes longer to find its way through the holey material.
So scientists use PCR to make glowing DNA copies of 13 different STRs. The glowing DNA is then pulled through the holes in the capillary electrophoresis system using electricity. The scientists then get a readout of how big all 13 pairs of STRs are in a certain DNA sample. They can identify someone from this DNA fingerprint.
This is how scientists can tell us apart. But how can they actually read the letters of that DNA? In other words, how can they sequence of a certain piece of DNA? By using many of the same ideas I talked about with forensics.
Sequencing DNA
As I said earlier, the letters of DNA are too small to see. So to read DNA, scientists had to come up with a way to see each letter in order. They do this by using different colored fluorescence and some copying proteins.
First off, scientists need to be able to tell the letters of DNA apart. Remember, DNA is made up of four different chemical letters called A, G, C, and T. When scientists sequence DNA, they tell the letters apart by giving them different colors. For example, T glows red, A glows green, C glows blue, and G glows yellow.
Now the scientists don't mark the DNA sample with these colors. What they do instead is make copies of the DNA using PCR and these colored letters.
Unfortunately, scientists can't really read a long string of glowing letters very well either. So, scientists needed to get a bit trickier.
What they do is add something extra to the colored letters that stops the DNA from being copied. This is called a chain terminator. When one of these colored letters gets put into a copied piece of DNA, the copying stops.
Of course, this isn't helpful if there are a lot of these poisoned letters around. Otherwise you'll only be able to add the first letter before the copying protein is stopped!
What scientists do is add just a bit of the colored letters to lots of normal letters. If we had a very powerful microscope, the mix might look like this:
Notice how only some of the letters are colored. Because so few are, odds are that most of the copied DNA won't be stopped. But a small bit will. If we use our microscope again, we end up with this kind of mix:
Notice that the different DNAs have stopped at different places. The next step is to wash away all the unused polymerase, DNA letters, etc. Now we'll only be able to see the pieces of DNA that end in colored letters. Here is what our mixture might look like now:
The next step is for this mixture to be sent through the sequencing machine. Here is a simple version of what this might look like:
The DNA is sent through the holey material with the shortest copies moving through fastest. The sequencer records the glowing colors in order. For our mix, it would see green, green, red, blue, yellow and decode that into the sequence AATCG.
Of course this is a very simple example and I've left a number of steps out. But this is the basics of how a sequencer works. Basically a scientist copies, labels and then reads the DNA.
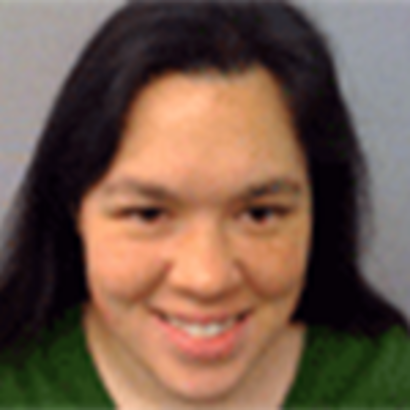
Author: Evonne Leeper
When this answer was published in 2009, Evonne was a Ph.D. candidate in the Department of Genetics, studying transcriptional regulation in cell type determination in Rick Myers’s laboratory. Evonne wrote this answer while participating in the Stanford at The Tech program.